The views expressed in this paper are those of the writer(s) and are not necessarily those of the ARJ Editor or Answers in Genesis.
Abstract
The question of how distant starlight can be seen in a young Universe has received much attention within creationist research. But creationist cosmological models need to explain much else in addition to the passage of light across vast distances. On both large and small cosmic scales there is a diverse range of trends, patterns, and phenomena that beckon some kind of explanation. Many of these can be understood plausibly within the framework of the standard “big bang” cosmology. But few attempts have been made to integrate them into a model for a young Universe. After surveying the astronomical evidence I discuss various avenues that creationist cosmology could profitably pursue in facing this challenge.
Keywords: cosmology, galaxies, young universe, distant starlight
Introduction
How can we see galaxies that are several billion light-years away if the Universe is only a few thousand years old? Or how could Adam have seen stars that were a few light-years away when the Universe was only a few days old? These are obvious questions for people to ask when considering the young-age creation position, and creationist writers have given much attention to them.
Advocates of a young universe have had to seek creative solutions to this distant starlight problem. For example, it has been suggested that the light was created in transit (for example, DeYoung 2010), that the speed of light may have been much greater in the past (Norman and Setterfield 1987), that Einstein’s general relativity with appropriate boundary conditions (Humphreys 1994, 1998, 2007, 2008) or with appropriate extensions (Hartnett 2007) can accommodate a young Universe, and that the problem itself assumes an arbitrary choice of convention for synchronizing clocks (Lisle 2010; Newton 2001).
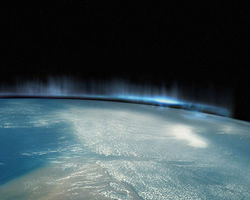
This focus on the light-travel-time issue can give the impression that, once this problem has been resolved, there are few, if any, significant challenges remaining for young-universe cosmological models. However, this is not the case; even if it is assumed that the distant starlight problem has been solved, there is much more that a young-universe cosmological model would need to explain.
The purpose of this paper is to consider those next steps for creationist cosmological models, beyond the problem of distant starlight. This builds on the work of Faulkner and DeYoung (1991) and Faulkner (1993, 1998), who argued that creationist models for astronomy need to address seriously the topic of stellar evolution.
After some discussion in the following section about what it means for a model to explain something, the next section contains a survey of various lines of astronomical evidence, along with their proposed explanations within a “big bang” cosmological framework (the “standard model” of cosmology; hereafter SMC). This is followed by an overview of some proposed creationist models for cosmology, with an evaluation of how well those models can explain what is seen in the universe. Finally, I conclude by discussing the implications for the current state of creationist cosmology.
What is an Explanation?
The task below will be to find explanations for observed astronomical phenomena: those trends and correlations that go beyond mere chance occurrences. But before attempting this, it is important to make clear the criteria that a legitimate explanation will be required to satisfy. We will denote the proposed explanation by E and the phenomenon (data) to be explained by D. There are various criteria that could be mentioned, but only one will be directly relevant to this paper.
The requirement is as follows: for E to be an explanation for D, it must be the case that D follows necessarily from E. This is one characteristic of the covering law model of scientific explanation (Hempel and Oppenheim 1948). Thus if D is the observation that a particular glowing light-bulb is generating heat, then an explanation for D would be E: “Whenever electricity passes through a resistor, some electrical energy is converted to heat, and this particular glowing light-bulb contains a resistor that is carrying an electric current.” In this case, D is a logical consequence of E, so E qualifies as an explanation for D.
Three points must be noted.
First, it follows that explanation is a stronger condition than consistency or accommodation. For example, the statement, E: “God created galaxies and God loves variety” can easily accommodate the observation, D: “most large galaxies have either spiral morphology or elliptical morphology”; the statement and the observation are entirely consistent. But, in this example, D does not follow logically or necessarily from E, so E does not qualify as an explanation for D. To express this in another way, an explanation E must be sufficient to answer the question, “Why do we observe D rather than not-D?” This is something we would intuitively expect from an explanation. But, in this case, E would not be sufficient to answer that question, because E is equally consistent with not-D, the (false) statement that “most large galaxies have morphologies that are neither spiral nor elliptical.”
Second, it should be noted that there can be, and often there are, more than one plausible explanation for a particular phenomenon. Hence, finding a plausible explanation, E, does not prove that E is the correct explanation.
Third, it should also be noted that there are different levels of explanation. At the highest level, we may seek an ultimate explanation for something, for example, for why something exists rather than nothing. A purely naturalistic cosmological model cannot satisfy this, whereas a creationist model does provide such an ultimate explanation. However, lower-level, proximate explanations are also of value. For example, a proximate explanation for a smashed window might be that it was struck by a brick. This is an acceptable explanation, even though it begs the answer to some higher-level questions: who threw the brick and why? This is relevant when evaluating proposed SMC explanations, which will necessarily be proximate rather than ultimate explanations.
Astronomical Phenomena that Require Explanations
The remaining tasks are to identify some astronomical phenomena that require (proximate) explanations, and then to seek explanations for these phenomena. Proposed SMC explanations will be considered in this section, while proposed creationist explanations will be considered in the following section. Alternative explanations have been put forward within cosmological models that are neither SMC nor biblical models; these will not be discussed in this paper. To simplify the discussion, the SMC will be treated as a “package deal,” with no attempt to dissect it into its constituent parts (including the “big bang” itself, inflation, dark energy, dark matter, and the formation of stars and galaxies). In addition, the focus will be on the successes of this model; this should not be interpreted as an attempt to hide the problems and weaknesses of the SMC, which are frequently discussed elsewhere in the creationist literature (for example, Williams and Hartnett 2005).
Oscillations in the cosmic microwave background
There is a faint background of microwave radiation reaching us from all directions in the Universe: the “cosmic microwave background” (CMB). It is widely known that this exhibits emission characteristic of a black body, i.e., something in thermal equilibrium, with a characteristic temperature of 3 K (Mather et al. 1994). What is less often mentioned is that this radiation also exhibits patterns in the variation of intensity with position on the sky. This is represented by the angular power spectrum of the CMB, which is shown in Fig. 1.
The general form of the CMB angular power spectrum can readily be explained by the SMC, and in fact was successfully predicted many years before it was observed (Peebles and Yu 1970). The proposed explanation is that the matter and radiation in the early universe behaved as a fluid in thermal equilibrium, in which the particles interacted through pressure as well as through gravity. Fluctuations in the density of this fluid would have travelled through the fluid as acoustic oscillations (sound waves). Just as a note played on a musical instrument has a fundamental harmonic and a sequence of harmonic overtones, so in this cosmic fluid, oscillations would have had a fundamental wavelength and a series of shorter-wavelength overtones. When the universe became transparent (with the radiation “decoupling” from the matter), the radiation would have retained this imprint of the oscillations. This would have shown up in the CMB as a series of peaks in the angular power spectrum, with a high peak at the fundamental oscillation scale, and a series of smaller peaks on smaller scales.
Detailed features in the clustering of galaxies
Galaxies cluster together. It is found that the clustering is stronger on smaller physical scales and weaker on larger physical scales. This transition from strong to weak clustering is smooth, apart from a few small oscillations, which were discovered in 2005 by teams working on two independent surveys of galaxy redshifts (Cole et al. 2005; Eisenstein et al. 2005).
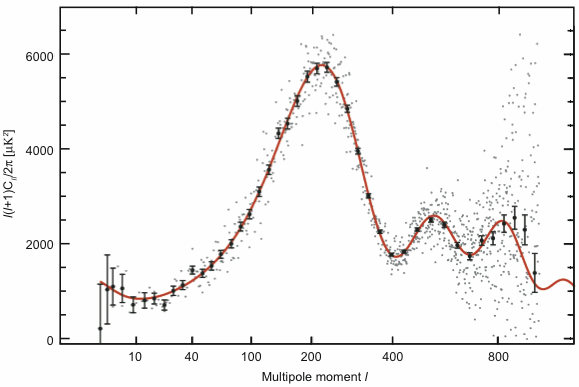
Fig. 1. The temperature angular power spectrum from the CMB, as measured by WMAP (after Dunkley et al. 2009). Angular scale is decreasing towards the right, with the highest peak corresponding to an angular scale of approximately 1°. The power spectrum shows that the greatest variation in CMB temperature happens on angular scales of a degree, with significant variation on scales of half a degree or a third of a degree. In the figure, the faint points show the individual data points, the darker points with error bars show the binned data, and the curve is a model fit to the data, where the model contains six parameters, which are tuned to produce the best fit to the data.
The SMC explanation for these oscillations is as follows. The ripples in the density of the cosmic fluid, seen in the CMB angular power spectrum (fig. 1), would persist to later cosmic epochs, leaving a slight imprint in how galaxies cluster. The best-fitting model for this, along with the observed data, is shown in Fig. 2. As with the CMB acoustic oscillations, this effect (known as the “baryon acoustic oscillations,” or BAOs) was predicted many years before it was observed (Peebles and Yu 1970).
The large-scale structure of the universe
Galaxies are observed to cluster together, forming a vast cosmic web with clusters, filaments, and voids. This is shown in the top and left panels of Fig. 3.
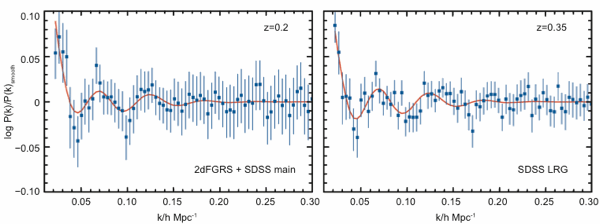
Fig. 2. Fluctuations in the power spectrum of galaxies, which quantifies the strength of galaxy clustering on different scales. The overall (smooth) power spectrum has been subtracted to show the oscillations (after Dunkley et al. 2009). The points with error bars are the measured values, and the lines are the direct predictions of the model fit in Fig. 1.
Within the SMC, this is explained as the outworking of the laws of gravity on small inhomogeneities in the density of matter over long periods of time. Computer simulations have been performed to test this. Particles of matter are placed randomly in a cube, with clustering properties consistent with the observed angular power spectrum of the CMB. These are then allowed to move under the influence of gravity. Images from one of these simulations are shown in the bottom and right panels of Fig. 3.
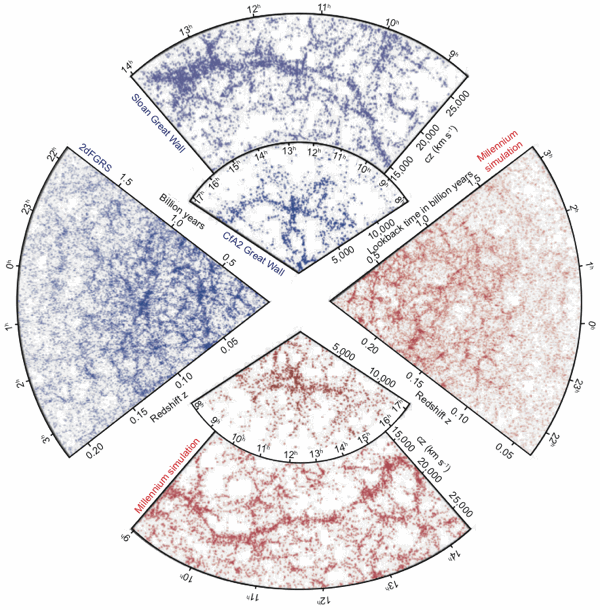
Fig. 3. Large-scale structure of the universe, in observations and simulations (after Springel, Frenk, and White 2006). The top and left panels show the observed positions of galaxies for three galaxy redshift surveys. The bottom and right panels show equivalently-sized segments from the Millennium Simulation, chosen to demonstrate that the same kind of structures are found in both observations and the simulation.
Galaxy properties and environment
Large galaxies can be classified according to their color and according to their morphology, with most being either red elliptical galaxies or blue spiral galaxies. In the nearby Universe, both color and morphology have been observed to exhibit strong trends based on the environment, with a greater fraction of elliptical galaxies and a greater fraction of red galaxies in higher-density regions (Bamford et al. 2009).
The SMC explanation for these observations is that these patterns reflect the dependence of galaxy formation on environment. For the dependence of color on environment, blue colors are taken to signify recent or current star formation, since the light from young stellar populations would be dominated by luminous high-mass stars with high surface temperatures. Star and galaxy formation would occur more rapidly in high-density environments, so galaxies in these regions would have finished forming stars several billion years ago. This means that galaxies in high-density environments would contain only old stars, and would be expected to be red in color. For the dependence of morphology on environment, galaxy–galaxy interactions would be more commonplace where the density of galaxies is higher, and interactions between galaxies would be expected to make the galaxies more elliptical in morphology, while also removing the gas reservoirs from the outer regions of the galaxies, thus preventing further disc and spiral formation from infalling matter.
Interacting galaxies
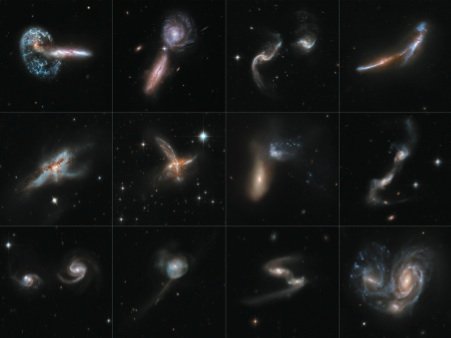
Fig. 4. Images of interacting galaxies taken by the Hubble Space Telescope.
Many galaxies are seen in close proximity, apparently interacting with each other, with greatly disturbed and entangled morphologies. Examples are shown in Fig. 4.
The SMC explanation for this is that the galaxies are indeed interacting, and in many cases are merging together into one larger galaxy. This would be expected, assuming galaxies interact through gravity, and assuming they do so over sufficiently long periods of time (many millions of years).
Structure of galaxies
Spiral galaxies are observed to have complex structures, with a disc, a stellar bulge, a stellar halo, and with different kinds of stellar populations found in these different components (Freeman and Bland-Hawthorn 2002).
The SMC explanation for this is that these features reflect the long process of galaxy formation and evolution. For example, star formation would occur predominantly in the disc of a galaxy, where the gas and dust reside, and the observable signatures of this would include (1) the presence of dust and gas in the disc, (2) bluer stars, and (3) higher-metallicity stars (that is, more heavy elements), compared with the rest of the galaxy. These signatures are all seen in the Milky Way Galaxy (Freeman and Bland-Hawthorn 2002).
Streams of stars in the Milky Way Galaxy
In mapping the positions of stars in the Milky Way Galaxy, astronomers have identified various distinct streams of stars, spanning large distances. An image showing some of these streams is shown in Fig. 5.
The SMC explanation for this is that the Milky Way Galaxy (as any large galaxy) has “swallowed” many smaller galaxies during its lifetime. The remains of some of these smaller galaxies would still be observable as distinct streams of stars in the Galaxy.
Clusters of stars
The Hertzsprung–Russell (H–R) diagram for a population of stars shows the surface temperature of the stars compared with their luminosity. For clusters of stars, either open clusters or globular clusters, the location of the stars on the H–R diagram forms a characteristic shape, with most stars lying on a “main sequence” and with a clear “turnoff” from the main sequence at a certain point. This is shown in Fig. 6.
The SMC explanation for this is that stars would form together in clusters, and that a population of stars that formed at the same time would display a clear turnoff point in its H–R diagram, with the position of the turnoff depending on the age of the population (Faulkner and DeYoung 1991; Kraus and Chaboyer 2003). Thus globular clusters would be considerably older than open clusters, having turnoff points further down the main sequence.
One other feature of globular clusters worth highlighting is the presence of “blue stragglers”: stars with anomalously blue colors, suggesting an age much younger than the apparent age of the cluster. This presents a challenge to the standard interpretation of globular cluster ages. However, their existence can tentatively be explained within the context of the SMC. As noted by Bernitt (2002), “By far the most preferred explanations today are ones that increase the mass of a star long after the cluster originally formed. In this way, the star can be old and blue at the same time.”
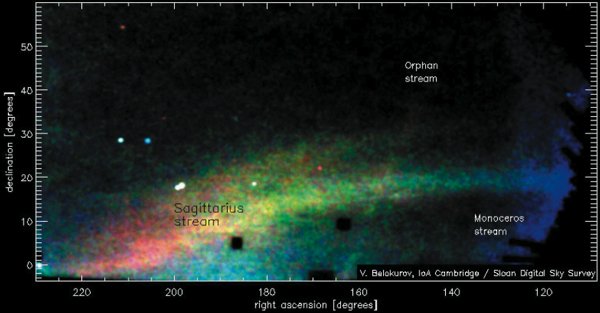
Fig. 5. Streams of stars in the Milky Way Galaxy (after Belokurov et al. 2006 a, b).
Seeking Creationist Explanations For These Phenomena
We have seen so far that there are many patterns and trends in the universe that beckon some kind of explanation, and that many of these appear to have plausible explanations within the SMC. However, even if plausible explanations exist, these explanations are not necessarily the only plausible explanations, or the best, so we now turn to seek alternative explanations for these phenomena, from a creationist perspective.
Special creation
The idea of special creation, most simply understood, is that the Universe we see is the universe God created ex nihilo on Day 4, and that it has not changed significantly since that time. This could be used to accommodate all of the phenomena mentioned in the previous section: God simply made the universe that way.
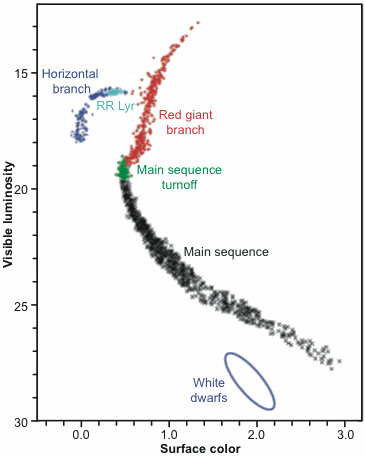
Fig. 6. An observed H–R diagram (color–magnitude diagram) for a typical globular cluster (after Kraus and Chaboyer 2003). The surface color corresponds to the surface temperature, with blue (hot) to the left and red (cool) to the right. The “Visible luminosity,” more commonly the “magnitude,” is an astronomer’s measure of brightness, with increasing magnitude corresponding to decreasing brightness.
However, this idea does not actually explain any of those phenomena, in the way explanations were described earlier. Moreover, the idea of special creation does not explain why the SMC has had so many successes.
Two specific forms of this idea of special creation are the creation of light in transit (DeYoung 2010; Whitcomb and Morris 1969, p. 369) and a model using an alternative convention for synchronizing clocks (Lisle 2010; Newton 2001), under both of which the universe we see is the universe essentially as it was when God created it 6,000–10,000 years ago.
Functioning creation
When Adam was created, he would have been a fully functioning human being. But this would require him to have some “apparent age,” since he would have been a “fully-grown” man. It has been suggested that the universe is similar, displaying “apparent age” in order to function properly.
This in turn begs the question of what the function of the universe is. For this we need to turn to Scripture. One purpose of the heavenly bodies is “to give light on the earth” (Genesis 1:17*); another is to “declare the glory of God” (Psalm 19:1). This suggests, among other things, that a functioning cosmos should have a certain size, grandeur, and stability.
However, it is difficult to see why the universe should need to have any of the specific properties described above in order to function in this way. For example, why does the universe need to contain interacting galaxies in order to function? Answers to such questions may be revealed after further investigation, but on the basis of our current understanding there is little reason to believe this approach could explain all of the phenomena above.
In response to this, it could be argued that the universe ought to be arranged so that God’s hand is not immediately apparent, noting that God is “a God who hides himself” (Isaiah 45:15) and “catches the wise in their craftiness” (Job 5:13; 1 Corinthians 3:19). Thus God could have specially created a universe that displayed properties consistent with a naturalistic origin, such as that proposed in the “big bang.” However, this is similar to the suggestion by Philip Gosse (1857) that the fossil record was specially created by God in the creation week, and raises the same theological questions about whether God would create something with such an apparent, but false, history.
Faster speed of light
It has been suggested that the speed of light was much greater in the past, and that a greater speed of light would enable us to see distant galaxies in a young universe (Norman and Setterfield 1987). While this could conceivably explain some of the phenomena above, such as certain properties of stars, there are some phenomena that depend clearly on the speed of matter, rather than the speed of light, such as interacting galaxies (Taylor 2005). In order to accommodate this, a varying speed of light would need to be coupled with additional components, such as processes taking place on very small physical scales prior to a rapid expansion of the universe (Setterfield 2006).
Time dilation
The idea that time may have run at different rates in different places in the universe (“time dilation”) is a central feature of some recent creationist cosmological models (Hartnett 2007; Humphreys 1994, 1998, 2007, 2008). This is used to solve the distant starlight problem, and further investigation into these models may conceivably lead to explanations for some of the phenomena described above. For example, many of the observations relating to galaxies and their stellar populations could be explained by a model that has billions of years passing within those galaxies before the light that we see was emitted.
However, difficulties occur when considering the Milky Way Galaxy. One can simplistically imagine that billions of years may have passed in the “distant universe,” while only thousands of years have passed in the “nearby universe,” but where is the transition between the “distant universe” and the “nearby universe”? For example, if the “nearby universe” includes the Milky Way Galaxy, then how can we see starlight from the other side of the Galaxy (tens of thousands of light years away)? Or if the “nearby universe” is not much larger than our Solar System, then what happened to the Solar System during the evolution of the rest of the Galaxy? Any transition scale between these two scales would presumably lead to large distortions in the Milky Way Galaxy, which are not observed. We are led to consider the earth or the Solar System suspended (supernaturally?) in a time dilation “bubble,” orbiting the Milky Way Galaxy for several billion years, before being released from this state presumably during the Creation Week. This is not something that has yet been addressed within creationist models of time dilation.
Old creation
The possibility that the universe might be genuinely old is not one that has received much consideration from within the creationist community. There is no logical contradiction between an old age for the universe and many core elements of creationism, such as a recent supernatural origin for life in the past few thousand years, the goodness of the original creation, death as a consequence of the Fall, and a recent global catastrophic Flood, which led to the formation of much or all of the fossil record. Attempts have been made to formulate such a “young biosphere, old universe” position (Gray 2009), but the biblical and theological consequences need careful assessment, and so far such attempts have tended to result in unusual and unnatural interpretations of Scripture (DeRemer 2005).
New models
A plausible young-universe explanation for the cosmological data may be something that will come at a future date. We learn from the history of science that many seemingly insurmountable problems have been solved by means of novel and creative ways of thinking. It may be that a creationist understanding of the cosmos will prove to be another example.
Conclusion
What is the current state of creationist cosmology? The brief survey in this paper would suggest that much work still needs to be done. Indeed, beyond the distant starlight issue, very little has been done to explain the many patterns and trends that have been identified through observations of the universe beyond our solar system. In contrast, while it does not supply an ultimate explanation, the SMC does apparently provide plausible proximate explanations for all of the phenomena discussed above.
The implications of this need careful consideration. It should be noted that a model’s ability to explain the observations can be misleading; for example, a model for the non-miraculous origin of the wine at Cana might be better than the true, supernatural, model at explaining the details of the chemical composition of the wine served at the wedding feast. But explanatory power is generally a good indicator that a model contains elements of the truth. The explanatory power of the SMC is itself something that beckons an explanation.
This has been a brief and selective sketch of some of the issues, but I hope future research will continue along these lines, seeking to find a cosmological model that is both faithful to Scripture and that has high explanatory power.
References
Bamford, S. et al. 2009. Galaxy Zoo: the dependence of morphology and colour on environment. Monthly Notices of the Royal Astronomical Society 393: 1324–1352.
Belokurov, V. et al. 2006a. The field of streams: Sagittarius and its siblings. Astrophysical Journal Letters 642:137–140.
Belokurov, V. et al. 2006b. Multiple galaxy mergers continue in the Milky Way. Retrieved September 23, 2010, from http://www.sdss.org/news/releases/20060508.mergers.html.
Bernitt, R. 2002. Globular clusters and the challenge of blue straggler stars. Journal of Creation 16, no. 2:5–7.
Cole, S. et al. 2005. The 2dF Galaxy Redshift Survey: power-spectrum analysis of the final data set and cosmological implications. Monthly Notices of the Royal Astronomical Society 362:505–534.
DeRemer, F. 2005. Young biosphere, old universe? A review of The age of the universe: What are the biblical limits? 2nd ed. by Gorman Gray. Journal of Creation 19, no. 2:51–57.
DeYoung, D. B. 2010. Mature creation and seeing distant starlight. Journal of Creation 24, no. 3:54–59.
Dunkley, J. et al. 2009. Five-year Wilkinson Microwave Anisotropy Probe observations: Likelihoods and parameters from the WMAP data. Astrophysical Journal Supplement 180:306–329.
Eisenstein, D. J. et al. 2005. Detection of the baryon acoustic peak in the large-scale correlation function of SDSS luminous red galaxies. Astrophysical Journal 633:560–574.
Faulkner, D. R. 1993. The role of stellar population types in the discussion of stellar evolution. Creation Research Society Quarterly 30, no. 1:8–12.
Faulkner, D. R. 1998. The current state of creation astronomy. In Proceedings of the Fourth International Conference on Creationism, ed. R. E. Walsh, pp. 210–216. Pittsburgh, Pennsylvania: Creation Science Fellowship.
Faulkner, D. R. and D. B. DeYoung. 1991. Toward a creationist astronomy. Creation Research Society Quarterly 28, no. 3:87–92.
Freeman, K. and J. Bland-Hawthorn. 2002. The new galaxy: Signatures of its formation. Annual Review of Astronomy and Astrophysics 40:487–537.
Gosse, P. H. 1857. Omphalos: an attempt to untie the geological knot. London: John Van Voorst.
Gray, G. 2009. The age of the universe: What are the biblical limits? 2nd ed., revised. Washougal, Washington: Morning Star Publications.
Hartnett, J. 2007. Starlight, time and the new physics. Eight Mile Plains, Australia: Creation Ministries International.
Hempel, C. G. and P. Oppenheim. 1948. Studies in the logic of explanation. Philosophy of Science 15:135–175.
Humphreys, D. R. 1994. A biblical basis for creationist cosmology. In Proceedings of the Third International Conference on Creationism, ed. R. E. Walsh, pp. 255–266. Pittsburgh, Pennsylvania: Creation Science Fellowship.
Humphreys, D. R. 1998. New vistas of space-time rebut the critics. Creation Ex Nihilo Technical Journal 12, no. 2:195–212.
Humphreys, D. R. 2007. Creationist cosmologies explain the anomalous acceleration of Pioneer spacecraft. Journal of Creation 21, no. 2:61–70.
Humphreys, D. R. 2008. New time dilation helps creation cosmology. Journal of Creation 22, no. 3:84–92.
Kraus, L. M. and B. Chaboyer 2003. Age estimates of globular clusters in the Milky Way: Constraints on cosmology. Science 299, no. 5603:65–69.
Lisle, J. P. 2010. Anisotropic synchrony convention—A solution to the distant starlight problem. Answers Research Journal 3:191–207.
Mather, J. C. et al. 1994. Measurement of the cosmic microwave background spectrum by the COBE FIRAS instrument. Astrophysical Journal 420:439–444.
Newton, R. 2001. Distant starlight and Genesis: conventions of time measurement. TJ 15, no. 1:80–85.
Norman, T. G. and B. Setterfield. 1987. The atomic constants, light and time. Menlo Park, California: Stanford Research Institute.
Peebles, P. J. E. and J. T. Yu. 1970. Primeval adiabatic perturbation in an expanding universe. Astrophysical Journal 162:815–836.
Setterfield, B. 2006. Response to “The Speed of Matter.” Retrieved March 23, 2010, from http://www.setterfield.org/GSRtaylorresponse.html.
Springel, V., C. S. Frenk, and S. White. 2006. The large-scale structure of the universe. Nature 440:1137–1144.
Taylor, J. K. 2005. The speed of matter. TJ 19, no. 3:91–95.
Whitcomb, J. C. and H. M. Morris. 1969. The Genesis Flood. London: Evangelical Press.
Williams, A., and J. Hartnett. 2005. Dismantling the Big Bang: God’s universe rediscovered. Green Forest, Arkansas: Master Books.